Inside Egypt’s Great Pyramid of Giza lies a mysterious cavity. This void has never been seen by any human alive today. Its surface is untouched by modern hands. Luckily, scientists are no longer limited to human senses.
To map out the pyramid’s unexplored interior, scientists tracked tiny particles called muons. Those subatomic particles are born high in Earth’s atmosphere.
From there, the muons hurtle toward the ground. Along the way, some have burrowed through the pyramid. Some of these particles left clues to their journey on sensitive detectors in and around the pyramid.
In 2017, the particles’ paths revealed the surprising presence of the hidden chamber.
That stunning find inspired physicists to explore other ancient structures the same way. The technique is now called muography (Mew-AW-gruh-fee). Some researchers are using it to map the inner plumbing of volcanoes. “You can see inside the volcano,” says Giovanni Leone. He’s a geophysicist at Universidad de Atacama. It’s in Copiapó, Chile. Such images could signal how and when a volcano is likely to erupt.
Muons form when high-energy particles from space — cosmic rays — crash into Earth’s atmosphere. Their smashups high in Earth’s atmosphere create a constant shower of muons. They rain down at various angles everywhere on Earth’s surface. Scientists are now looking to use them to peer inside structures anywhere and everywhere.
When the muons reach Earth’s surface, they tickle the insides of large structures. Such as those pyramids. (They zip through smaller stuff too. Your thumbnail is pierced by a muon about once a minute.) Measuring how many muons something absorbs as they pass through it can reveal how dense the structure is. That, in turn, can expose any hidden gaps in the material.
The technique is sort of like taking a huge X-ray, explains Mariaelena D’Errico. But “instead of X-rays, we use … a natural source of particles.” That is, Earth’s very own, never-ending supply of muons. D’Errico is a particle physicist. She works at the National Institute for Nuclear Physics in Naples, Italy.
In the past, physicists studied cosmic rays to better understand outer space. But muography turns this tradition on its head. It uses these cosmic particles to learn more about concealed parts of our own world.
For the most part, “particles arriving from the universe have not been applied to our regular lives,” says Hiroyuki Tanaka. This particle physicist at the University of Tokyo and others are trying to change that.
A particle like no other
Muons are like the awkward cousins of electrons. Like electrons, they carry a negative electric charge. But muons are much heavier than electrons. And, unlike electrons, they don’t play a key role in atoms. In fact, when muons were first discovered, physicists wondered why these strange particles existed at all.
Muons, it turned out, are ideal for imaging the insides of large objects. A muon’s mass is about 207 times as large as an electron’s. That extra bulk means muons can pass through hundreds of meters of rock or more. If an electron passes through matter like a bullet, a muon tears through like a cannonball. A wall may stop a bullet, while a cannonball can pass through.
Another upside of muons: They are plentiful. They rain from the sky everywhere, all the time. So muon imaging needs no artificial radiation beam, such as the one produced by that X-ray machine in a doctor’s office. Muons “are for free,” says Cristina Cârloganu. This particle physicist works at CNRS and the National Institute of Nuclear and Particle Physics. She’s based in Aubière, France.
“They’re also very easy to detect,” says Richard Kouzes. He’s a nuclear physicist. He works at the Pacific Northwest National Laboratory in Richland, Wash. A simple detector made of plastic strips and light sensors can pick up muons. Other detectors need little more than special photographic film. Such instruments can detect both muons and their antiparticles. “Antimuons” are like muons, but carry a positive charge. They, too, shower down on Earth from high in the atmosphere.
When muons and antimuons pass through an object, they lose energy in various ways. One is by colliding with electrons in the material. That energy loss slows the particles. Sometimes they even stop. The denser the material, the fewer muons and antimuons that make it through to a detector under or next to the material.
Large, very dense objects — such as volcanoes or pyramids — will cast a muon shadow. Any gaps within those structures appear as bright spots within the shadow (because more particles slipped through). Inspecting such dappled shadows can open a vista into hidden worlds.
Probing pyramids
Muography first proved itself in a pyramid. In the 1960s, a team led by physicist Luis Alvarez hunted for hidden chambers in Khafre’s pyramid in Giza. This monument is a slightly smaller neighbor of the Great Pyramid. Detectors found no hint of unexpected rooms. The search did, however, prove the technique works.
Still, its use took didn’t take off right away. Muon detectors of the era tended to be bulky. And they worked best in well-controlled labs. To spot muons, Alvarez and his team used detectors called spark chambers. These chambers are filled with gas and metal plates under high voltage. When charged particles such as muons pass through, they create trails of sparks.
Today tech has largely replaced spark chambers. “We can make very compact, very sturdy detectors,” says Edmundo Garcia-Solis. He’s a nuclear physicist Chicago State University in Illinois. One type of detector that works outside the lab contains a type of chemical known as a scintillator. It emits light when a muon or other charged particle passes through it. Electronics then capture and measure that light.
This year, physicists will use these detectors to take another look at Khafre’s pyramid. Kouzes and his colleagues announced their plan February 23 in the Journal for Advanced Instrumentation in Science. Their detector is small enough to fit inside two large carrying cases. Once inside the pyramid, it can be run with a laptop.
A nuclear emulsion film was key to finding the Great Pyramid’s hidden void in 2017. As muon detectors go, this muon detector is pretty low maintenance. It uses a special type of film to record the tracks as muons pass through. Researchers left detectors sitting in and around the pyramid. Later, they brought those films back to a lab to study the particle tracks they had recorded.
Kunihiro Morishima is a particle physicist at Nagoya University in Japan. He helped discover the Great Pyramid’s secret chamber. “Nuclear emulsions are lightweight, compact and do not require a power supply,” he says. That means multiple detectors can be placed at prime viewing sites. One of those was a room in the pyramid called the Queen’s Chamber. The team set up plastic scintillator detectors there, too. Meanwhile, gas-based detectors collected data from outside the pyramid.
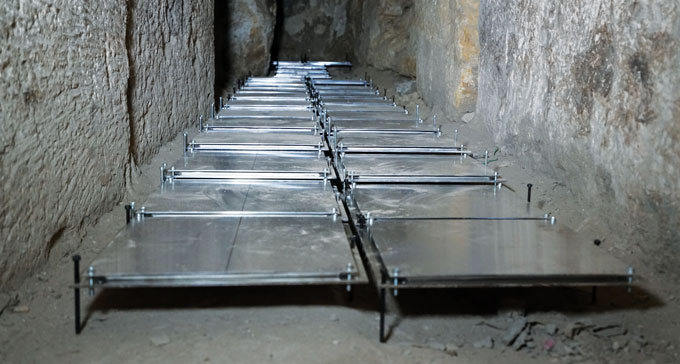
Since discovering the void, Morishima and his colleagues have been busy taking more measurements. These data have helped sketch details of the void. The team placed emulsion detectors at 20 locations in the pyramid. They also put gas detectors at different spots. This new array of instruments revealed that the void is more than 40 meters (131 feet) long. The purpose of this space remains unknown.
Another group is planning a bigger survey of the Great Pyramid. Their idea is to place much larger detectors outside the pyramid. The detectors will be moved from place to place to measure incoming muons from multiple angles. This should give a 3-D view of what’s inside, says Alan Bross. He’s a particle physicist at Fermilab in Batavia, Ill. He is part of a team that shared its plans March 6 in the Journal for Advanced Instrumentation in Science.
Pyramids elsewhere in the world are also getting closer scrutiny. Garcia-Solis at Chicago State University and his colleagues plan to use muons to probe El Castillo. It’s a Maya pyramid at Chichén Itzá in Mexico. Morishima’s group plans to work on Maya pyramids, too. Scientists hope such studies might reveal new chambers or other hidden features.
There are other ways to peer inside objects. Radar, for example. Or ultrasound. Even X-rays. But each of these can probe only a short distance from the surface. Muons, in contrast, can paint an in-depth picture. For studying pyramids, Bross says, “muons really are ideal.”
Peering inside a volcano
Vesuvius is a known menace to Naples and surrounding areas in Italy. The volcano famously destroyed the ancient city of Pompeii in A.D. 79. Another major eruption in 1944 destroyed nearby villages. Since then, the volcano has been quiet. But if it erupted today, it could endanger the lives of some 600,000 people around it. Such a disaster might also threaten many others somewhat farther away.
“Vesuvius always scared me,” D’Errico says. “I was born and I live under this volcano.” Now, D’Errico is part of the Muon Radiography of Vesuvius experiment — MURAVES for short. Through this work, D’Errico seeks to better understand the volcano and its threat.
The team has set up muon detectors 1.5 kilometers (not quite a mile) from the volcano’s crater. This network is mapping muon densities — and thus rock densities — at the volcano’s top. It’s already turned up hints of density differences between the volcano’s northwestern and southeastern sides. The team shared that finding February 24 at arXiv.org.
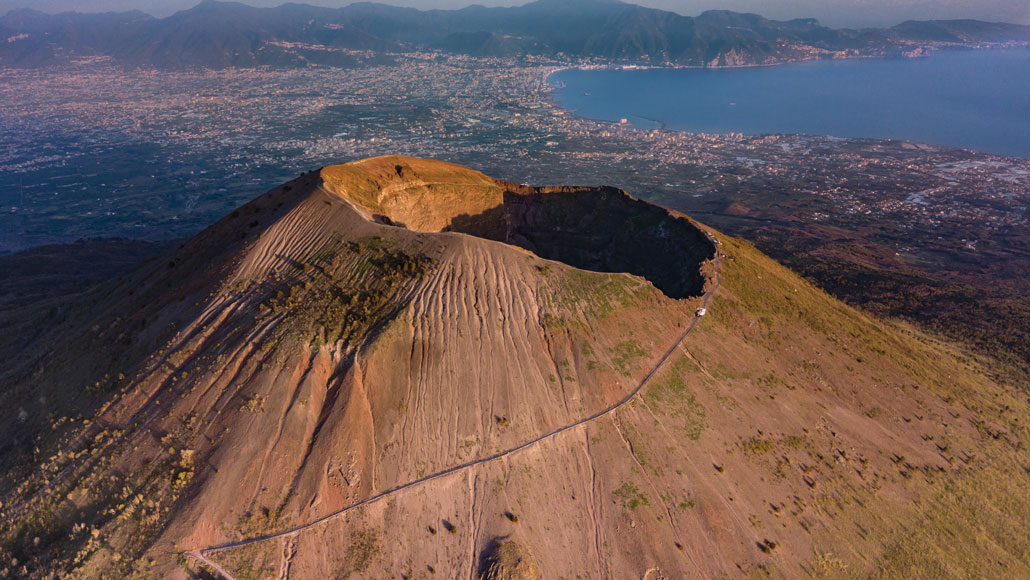
MURAVES is still collecting data. Future observations should help scientists better understand the finer details of the volcano’s structure. The insides of Vesuvius are thought to be layered from repeated eruptions. Each new one could have piled new material atop the old.
Understanding a volcano’s structure could help predict what will happen when Vesuvius erupts next. For instance, it might help forecast where landslides could occur. Planning for those risks might help keep nearby people safe, says Cârloganu.
To see how, just look at Mount St. Helens in Washington. An entire flank of the volcano collapsed in a 1980 eruption. The disaster killed 57 people and caused widespread damage. Knowing where a volcano is weak could help predict how an eruption might play out, Cârloganu explains. And that could indicate what communities sit inside a danger zone.
Cârloganu has used muons to study a dormant volcano near Clermont-Ferrand, France. Now, she’s working to image Italy’s volcanic island of Vulcano. She thinks muons could point out this volcano’s weaknesses. But she doesn’t think they would warn when the volcano is going to blow.
Other researchers are more optimistic. Leone and Tanaka are among them. They wrote a paper on the topic last November. Muon imaging is ripe to include in volcano early-warning systems, they said. But it will take work, they noted in the Proceedings of the Royal Society A. Muon imaging has to be combined with other proven methods of eruption-forecasting, Leone says. Those include seismic measurements. Observations of ground motion and volcanic gases play a role, too.
Tanaka and colleagues are currently studying one of the most active volcanoes in the world. Called Sakurajima, it’s near Kagoshima, Japan. The volcano’s Showa crater erupted often until 2017. Then, the activity shifted abruptly to a different crater, Minamidake. By comparing muon data from before and after this happened, Tanaka’s team may have discovered why the shift occurred. A new, dense region had formed below the Showa crater. So Showa may have stopped erupting when a dense mass of solid magma plugged the crater.
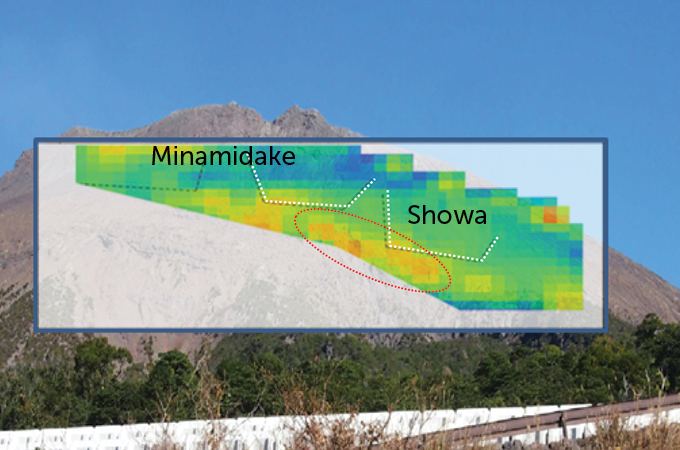
These results suggest that scientists can use muons to help predict eruptions, Tanaka says. And so does another of his recent studies. Here, Tanaka and his colleagues fed muon data on the volcano’s structure into a deep-learning system. (Deep learning is a type of computer algorithm that can learn to make predictions from data.) Based on the muon data from a given week, the deep-learning system could predict whether the volcano would erupt the next day. The system correctly predicted eruption days more than 72 percent of the time. It correctly predicted non-eruption days more than 85 percent of the time.
Physics has incredible power to change how we see the world around us. The discovery of X-rays unveiled a whole new way to see hidden depths. Now, harnessing muons could change our perspective again. Science is starting to truy appreciate a particle once thought to be unnecessary. One day, in fact, these muons might save lives.
#Physics | https://sciencespies.com/physics/muons-reveal-the-inner-worlds-of-pyramids-volcanoes-and-more/
No comments:
Post a Comment